It’s important to realise that the cat inside the box is both alive and dead, the physicist Erwin Schrödinger told a (presumably bemused) Albert Einstein in 1935. As a way of explaining the principle of quantum superposition, wherein any particle can possess two or more quantum states, Schrödinger imagined a cat – representing said atomic particles – inside a closed box with a Geiger counter and a flask of poison. If the dial on the counter begins to twitch at any point, the flask is broken and the poison presumably kills the cat. However, since the particles can exist in multiple states at once, the cat is actually both alive and dead until the box is opened, when one of either state is confirmed.
Such is the principle underlying how quantum computers work. Unlike a classical computer, in which bits that can either represent 0 or 1 are the basic unit of calculation, a quantum computer uses qubits, which can individually represent 0 or 1 simultaneously. This makes it much more efficient at processing complex calculations, but also incredibly hard to build. Not only is the stability of quantum computers dependent on running them at extreme conditions, including ultra-low temperatures (a recent working demonstration at -272°C was considered a breakthrough), there is also an inherent limitation to how many qubits can be harnessed by an individual machine.
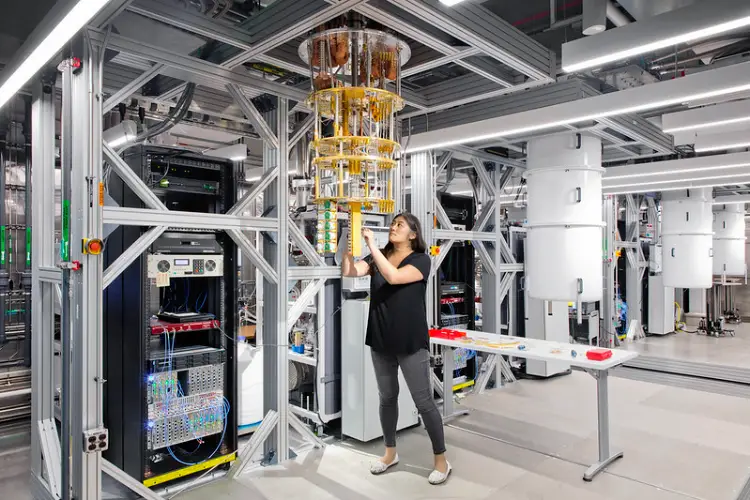
As quantum computers become more advanced, increasing attention is being paid toward networking them together. (Photo by IBM /Flickr)
As such, progress has been slow. The first two-qubit quantum computer was built in 1998. In December 2020, researchers in China got that up to 76 qubits. The resulting computer was immensely powerful, performing calculations trillions of times faster than some of the most advanced supercomputers. Even so, it still provided only an inkling of what quantum computers could achieve in a networked state. Achieving this, again, is extremely difficult. Quantum states are already immensely fragile, and the process of communicating quantum data down a wired connection has so far proved vulnerable to interference, a risk exacerbated by distance.
Earlier this month, however, a team from Toshiba managed to send a quantum message down a fibre-optic wire some 600km long. It was primarily a demonstration of Quantum Key Distribution (QKD), a method of secure communications that uses the principles of quantum mechanics to send a cryptographic key, mapped onto a photon, that is destroyed should anyone snoop on the message in transit. But the company hailed the breakthrough as a first step toward a quantum internet.
What is the quantum internet?
“The quantum internet is really a future development, or evolution, of these quantum communication systems that we have today, which will allow us to build a global network of quantum processors that are connected together by quantum communication links,” explains Andrew Shields, head of Toshiba Europe’s quantum technology division.
The quantum internet… will allow us to build a global network of quantum processors that are connected together by quantum communication links.
Andrew Shields, Toshiba Europe
Not only would this allow for secure communication networks, says Shields – QKD is perhaps the only cryptographic method that cannot be broken by quantum computers – but distributed quantum computing on an epic scale. “That would allow secure communications, as QKD does, but also many other things,” says Shields. These include solving vast mathematical problems beyond the bounds of classical supercomputers, with profound implications in everything from financial modelling and cybersecurity, to pharmaceutical research and astronomy.
Quantum internet applications
The quantum internet is unlikely to resemble the internet of today – a global communications network hosting everything from social media to encyclopaedias and e-commerce. “We shouldn’t think about [it] as something that replaces the classical internet,” says Tracy Northup, a professor in experimental physics at the University of Innsbruck. Rather, the quantum internet should simply be defined as a network “that would let you distribute quantum entanglement as a resource.”
For most researchers, the purpose of quantum communication is to enable distributed access to quantum computing. In that way, the quantum internet is likely to be closer to the pre-commercial days of the classical internet, in which two or three US university campuses shared data and computing resources.
That stage is yet to come for the quantum internet. Connecting quantum processors, either down a wired connection or through the air, requires several pieces of equipment that are still in the experimental stage. Imagine, for example, two quantum computers, one at Station A and the other at Station B. One of the most promising ways for them to talk to one another is by sharing particles that have been ‘entangled,’ meaning they share perfectly correlated quantum properties to the point that they act like mirror images of one another irrespective of distance. The easiest way to do this is to transmit photons, sometimes known as ‘flying qubits,’ between Stations A and B.
Several factors make this operation fiendishly difficult. For one thing, transmitting the qubit in this way makes it vulnerable to interference or ‘noise,’ which risks compromising the integrity of the message. “If we lose that information on the way, or if it gets distorted, we have to live with that distortion,” explains Northup.
In classical communications, this problem is solved by using a repeater, explains Shields, “which can amplify the optical signal and allows you to have communications over any arbitrary distance”. A similar device can perform the same role within a quantum network. “A quantum repeater will expand communication to any arbitrary distance,” says Shields, far in excess of the 600km record set by Toshiba earlier this month.
Indeed, a few days before the company’s breakthrough, a team from the Institute of Photonic Sciences (ICFO) in Barcelona, Spain, announced their own breakthrough, ‘a crucial step towards a viable realisation of a quantum repeater.’ The team succeeded in sending an entangled photon from one laboratory to another some ten metres away, storing and recovering it from a memory device along the way. “They achieved over 90% fidelity, which is extremely good,” says Myungshik Kim, a physics professor at Imperial College London. “But, if you ask me if that’s good enough for a good quantum internet, then I would think we should improve more.”
For the quantum internet to avoid non-negligible error rates, that entanglement would need to be boosted to 100%. Even so, part of what makes the Barcelona paper so exciting is that it is theoretically possible to refine the entanglement the team achieved into something even purer. “We can distil an imperfect entanglement to 100% maximally entangled pairs,” explains Kim. That is likely to be the next challenge to overcome in quantum teleportation. “We need a few more years to go” before we begin to see the fruits of that research, says Kim.

Networking individual processors into a 'quantum internet' could help increase computational power. (Photo by Craig Raymond/Shutterstock)
Is the quantum internet unhackable?
While the quantum internet is not yet a reality, though, its foundations are already in use. “Quantum key distribution for quantum communication is already a mature technology that’s being commercialised,” says Northup, used to secure data on everything from bank transfers to Swiss election results. Its selling point as a secure communication method theoretically immune from hacking even by quantum computers has made it ideal for encrypting data that needs to remain inviolable indefinitely.
“We did a trial in Sendai, Japan, which involved securely communicating genome data,” explains Shields, “data that we have to keep secure for many years over a person’s lifetime, and maybe even the lifetime of their descendants, as well.”
This is not to say it is completely immune from hacking. Researchers like Dr Vadim Makarov at the Russian Quantum Center in Moscow have been busy for more than a decade in finding ways to disrupt or crack quantum cryptographic systems. “What ‘quantum hackers’ have really emphasised is that this method of secure communication will only work if you trust the physical implementation,” says Northup. Getting around this has led to the creation of an entirely new field: device-independent quantum key distribution (DIQKD), a series of tests touted by its proponents as ‘the ultimate form of cryptography,’ but one that Shields regards with skepticism (“It is not possible to build any cryptographic system, quantum or classical, without some assumptions about the equipment,” he says. “DIQKD does not change the situation.”)
What ‘quantum hackers’ have really emphasised is that this method of secure communication will only work if you trust the physical implementation.
Tracy Northup, University of Innsbruck
Even so, the popularisation of QKD augurs a new era for secure communications. What implications this will have on policing and intelligence, let alone personal and corporate privacy, is unknowable. Neither is the ultimate impact of the quantum internet, the locomotive for which the infrastructure supporting QKD will be so many tracks and sleepers.
That uncertainty hasn’t stopped investment pouring into research projects investigating the potential of the quantum internet around the world. “You’re also starting to see companies get involved,” says Northup, all of which is providing “a really rich environment for people to look at these questions and to collaborate.”
Not only has this led to a series of quantum networking breakthroughs in quite remarkable succession, but also an increasing interest in precisely what applications could result when the quantum internet is finally realised. Just how researchers may bring to bear the vast computing power cannot be foreseen, in much the same way that the scientists experimenting with the early classical internet could never have known it would lead to the mass sharing of information on a global scale. Even so, as we enjoy the fruits of quantum research, says Northup, we must not forget how they were originally sown.
“I would like to highlight the continued importance of basic research,” says Northup, pointing to the fact that several of the ideas coming to the fore in quantum networking – most notably DIQKD – are incredibly new. “I worry that… it may be easy for people to picture it all as an applications question, about how to build it, test it, get the construction done. But I think the basic scientific work will continue for a long time to play a very important role.”