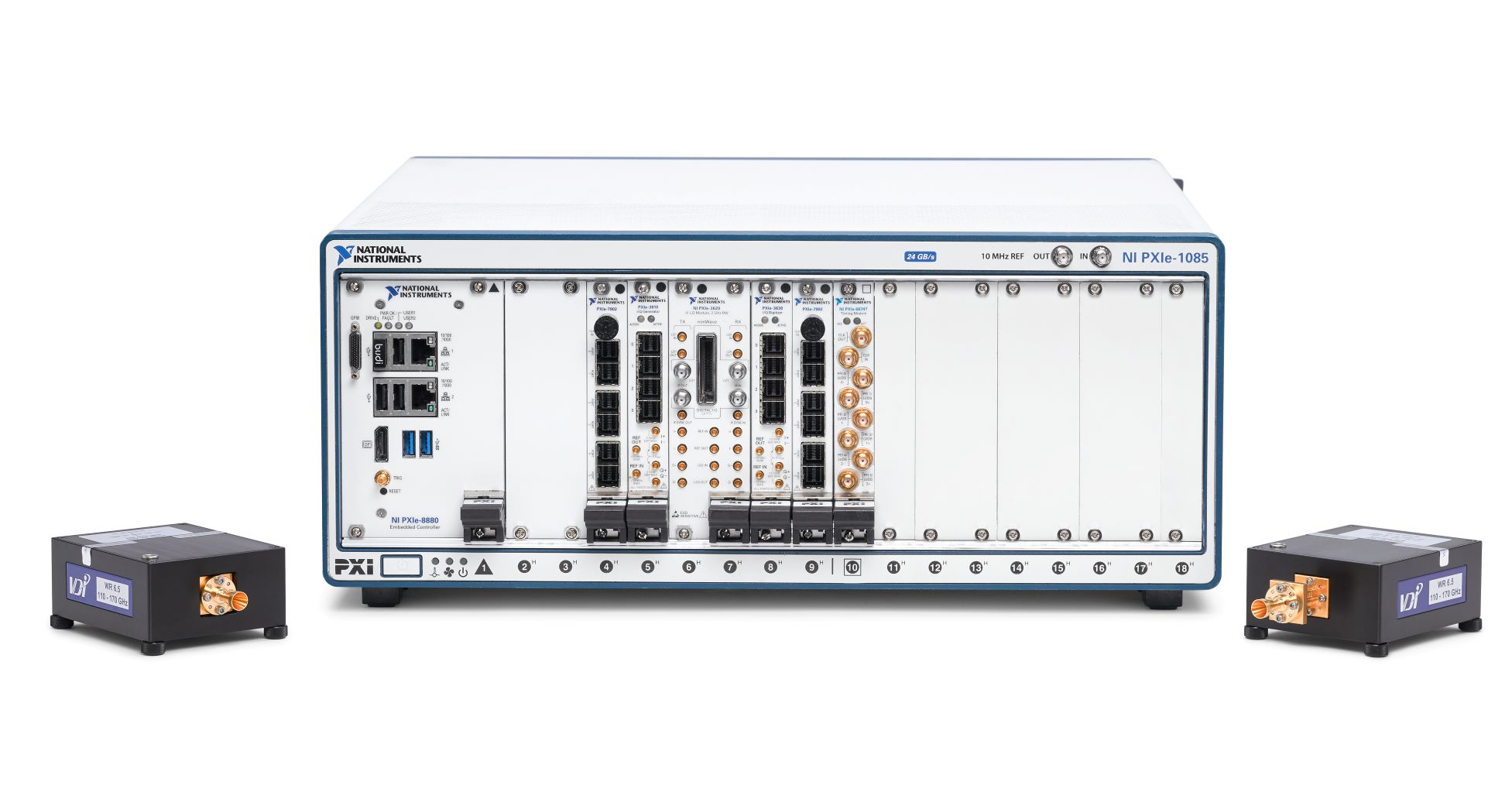
The world of 5G is barely beginning to be explored by businesses – its advocates tout a vision of smart factories; mobile game streaming; AR-augmented surgery; autonomous vehicles, and Pokemon Go on steroids – with network carriers rolling it out in phases this year and into 2020 in the UK, but experimental 6G work is already starting.
Texas-headquartered National Instruments (NI) gives a flavour of what that might look like this week, with the release of a sub-THz software defined radio (SDR) for 6G research, built on its mmWave Transceiver System and Virginia Diodes’ radio heads.
(The development cycle of a typical wireless standard is approximately 10 years, it notes; with 5G roll out gaining pace this year, 6G could emerge by 2029…)
Sub-THz, You Say?
With even 5G still rather more contested a term than it should be, what 6G might constitute is, at this stage, something of an open question.
Bets are being placed, however, on the use of the Terahertz (THz) frequency range (0.1 THz — 3 THz); the last span within the electromagnetic wave spectrum. (THz typically refers to 0.1–10 THz; sub-THz region is 0.1–0.3).
NI’s new gizmo, which is built on FPGAs (customisable and programmable chips) can be upgraded and customised to create a “real-time testbed for 6G research”, the company says, and is equipped with special radio heads that that can transmit/receive a wide variety of frequency bands in the sub-THz range.
Read this: Industry-First Xilinx Accelerator Card Runs in “Any Server, Any Cloud” – Fits Standard PCIe Slots
“Researchers need access to sub THz testbeds to prototype multiple wireless use cases. These testbeds must be highly flexible but also offer cutting edge performance in order to explore the boundaries of wireless performance at these very high frequencies,” said James Kimery, director of wireless research, NI.
He said the offering will “spur innovation on the way toward 6G.”
All signal processing, including coding, occurs in real-time on FPGAs added to the base transceiver system, the company said in a release today, with total system throughput is dependent on the frame structure and the number of channels used.
A typical expected throughput is 7.2 Gbps/channel, with a software front panel offering real-time visualisation of system level performance.
Can sub-THz/6G Networks… Work?
With massive MIMO-based 5G already a challenge for engineers (signals are easily block by physical structures, etc.) sub-THz/6G networks look set to require some ingenious materials developments.
As academics from Saudi Arabia’s King Saud University and France’s IETR University of Rennes put it in a joint paper, “Sub-THz Antenna for High-Speed Wireless Communication Systems” published March 2019: “Very high path loss is imposed as one of the main challenges at THz band frequencies, which poses a major constraint on communication distances.
“Additional challenges range from the implementation of compact high-power THz band transceivers, the development of efficient ultra-broadband antennas at THz Band frequencies, and characterization of the frequency-selective path loss of the THz band channel to the development of novel modulations, transmission schemes, and communication protocols tailored to the peculiarities of this paradigm.”
Other specialists at the coalface of network R&D agree.
“Admirable output powers, but only at cryogenic temperatures”
In a June 2019 paper “A Perspective on Terahertz Next-Generation Wireless Communications“, Oklahoma State University academics John F. O’Hara, Sabit Ekin, Wooyeol Choi and Ickhyun Song noted that the generation, reception, and conversion of terahertz waves in mobile devices requires “cutting-edge electronic, photonic, or hybrid approaches that push the limits of material properties and device capabilities.”
Modern photonic-based THz sources include quantum cascade lasers (QCLs); nonlinear optical-mixing sources, and ultrafast laser-driven pulsed sources and detectors.
They note: “The form factors and operational principles of these vary so widely that one-to-one comparisons in performance are very challenging. For example, terahertz QCLs can achieve admirable output powers, but only at cryogenic temperatures, whereas mid-infrared QCLs, used in conjunction with nonlinear crystals can make microwatts of tunable continuous wave (CW) terahertz (1-5 THz) at room temperature…
“All of these sources offer impressive performance in their own ways, but none so far
are easily integrated into larger digital electronic systems, which is arguably their biggest downfall for communication systems.”